This is the third piece in a joint series by Employ America and the Institute for Progress called Hot Rocks: Commercializing Next-Generation Geothermal Energy. We’re examining the potential to commercialize next-gen geothermal energy, the lessons we might learn from the shale revolution, and the federal policy changes needed to make it happen. The introduction to the series is available here. In our first piece, Brian Potter illuminated the incremental technological innovations that led to the shale revolutions. In our second piece, Arnab Datta and Skanda Amarnath described key policy decisions that facilitated the private capital investment necessary to fund and scale those innovations.
The promise of next-gen geothermal
Next-generation geothermal energy is poised for a takeoff. The heat energy stored in Earth’s crust is massive, more than the energy in all known fossil fuels. For decades, this energy source has been hard to exploit at a scale large enough to compete in global energy markets. But the same advances that birthed the shale revolution have created strong tailwinds for next-gen approaches to tapping geothermal resources.
Getting to a world with abundant geothermal will require continued technological innovation and widespread commercialization. Next-gen geothermal companies need a friendly policy environment to facilitate investment. That investment is necessary to produce technological advancements and move down the cost curve.
Below, we take stock of the current state of next-gen geothermal, the technological challenges needed to achieve commercial scale, and why the current capital and regulatory environment falls short.
The technological barriers to next-gen geothermal
Reaching the thermal energy in earth’s crust is fundamentally a technological problem. Conventional geothermal systems (sometimes called hydrothermal) have historically been constrained by the limitations of drilling technology and traditional production techniques. Technological limitations have forced conventional geothermal producers to rely on three highly specific naturally occurring geological conditions: heat, fluids, and permeability. To access geothermal heat with limited drilling technologies, conventional systems have to find the rare locations where heat is close to the surface, like near the intersection of tectonic plates. Conventional systems also require natural reservoirs with high permeability where existing fluids easily flow between injection and production wells. Only once all three of these factors are located can conventional wells efficiently recover geothermal energy: by pumping fluids down an injection well, recovering heated fluids from a production well, and converting the heat into electricity by spinning a turbine.
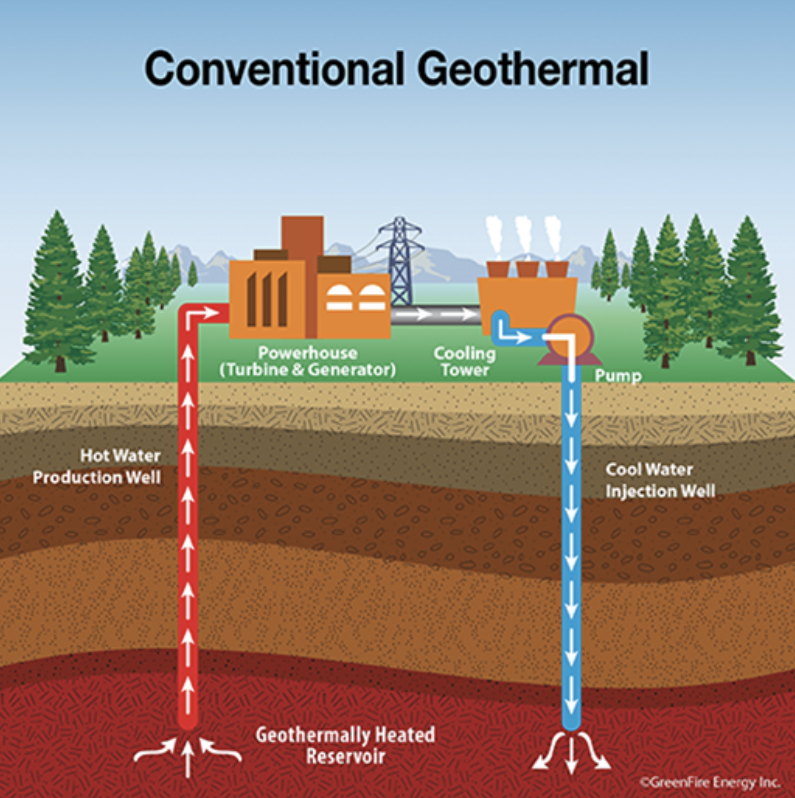
Relying on specific, naturally-occurring geological factors heavily limits the scale of conventional geothermal energy. Heat, fluids, and permeability rarely come together naturally. The result is that just 0.4% of the United States’ electricity production comes from geothermal.
Next-gen geothermal approaches use technological advances to bypass the constraints of conventional systems. Several companies are using innovations from the shale revolution to access the crust’s abundant heat by creating artificial reservoirs. Others are going all in on the future of deep drilling, looking to access heat in super deep “basement” granite.
Enhanced geothermal systems
Where conventional systems require natural reservoirs with high permeability, enhanced geothermal systems (EGS) designs use horizontal drilling and hydraulic fracturing to create artificial permeability. By breaking open fissures in the subsurface, they allow water to circulate between injection wells and production wells. Essentially, fracking creates the reservoir.
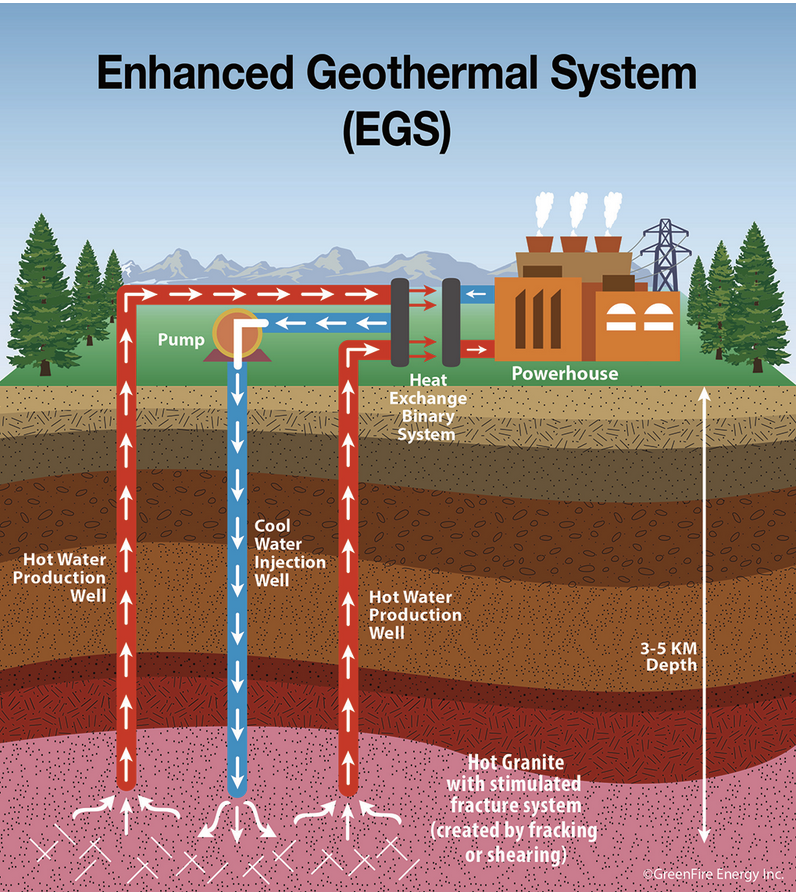
EGS had a recent breakthrough, as Fervo Energy completed a 30-day well test. By using a “radically different and more modern stimulation design,” Fervo was able to achieve flow rates higher than previously possible — up to 63 L/s, “equivalent to a peak gross electric power output of 3.5 MW.”
ResFrac explained the technical achievement and Fervo’s unique approach.
“The pursuit of a dense network of shear-stimulated natural fractures has led to stimulation designs that would be considered uneconomic if applied in an oil and gas setting – a single fracturing stage, a vertical well, and no proppant. Fervo applied the learnings of the shale industry to EGS.”
FERVO is already setting out to repeat and build on its success, announcing a 400 MW plant it hopes to bring online by 2028. DOE’s FORGE project is also studying techniques for commercially scalable EGS.
As Marc McClure of ResFrac stated, there are numerous technological barriers to overcome.
“To reach full potential, [FERVO] need to test longer wells and alternating series of producer/injectors (so that wells can flow in either direction). They need to do careful work to assess the optimal well spacing. Even as they continue to achieve technical success, they will need to demonstrate the commercial model – that they can secure funding, execute, and drive down costs to achieve long-term profitability.”
However, producing these wells currently costs far too much — over ten times more than other onshore renewable sources — to be commercially viable. The challenge now for EGS is scaling costly demonstration projects to affordable and widespread deployment. FERVO and others need to prove that recent breakthroughs can be repeated efficiently and that costs can come down. EGS needs to be able to handle different geological conditions and operate for longer periods of time. Production needs to continue to become more efficient and development needs to become more streamlined. EGS also needs to convince oil and gas companies that it is a more profitable alternative to shale fracking, or to simply disbursing dividends to shareholders.
Closed-loop systems
Closed-loop systems forgo the use of subsurface reservoirs, and instead create closed-off, sealed systems. Where EGS systems work through “convection,” fluids heated by large surface area reservoirs rise when colder fluids are injected into the system, closed-loop systems work purely via conduction: injected fluids are pumped through a pipe loop, are heated by conduction with the hot rocks, and then pumped back to the surface to generate electricity. According to a recent research paper presented to the Society of Petroleum Engineers,
“These solutions are attractive because they do not rely on the natural permeability of a formation or a reservoir of heated water already in place, they simply require a high enough downhole temperature.”
One upside of this approach is that in closed-loop systems, geothermal plants can use “supercritical fluids” rather than water as the main heat transfer fluid. A supercritical fluid is a substance at a temperature and pressure above its critical point, where distinct liquid and gas phases do not exist, but below the pressure required to compress it into a solid. At supercritical states, fluids can hold more energy per unit of mass. Some chemical compounds, like CO2, can convert to supercritical fluids at lower temperatures than water. Supercritical fluids are significantly more efficient at transferring energy per unit, leading to large increases in generation efficiency, as “wells discharging supercritical fluids could generate up to ten times more power than conventional geothermal boreholes.” Geothermal company Eavor is pioneering the closed-loop approach.
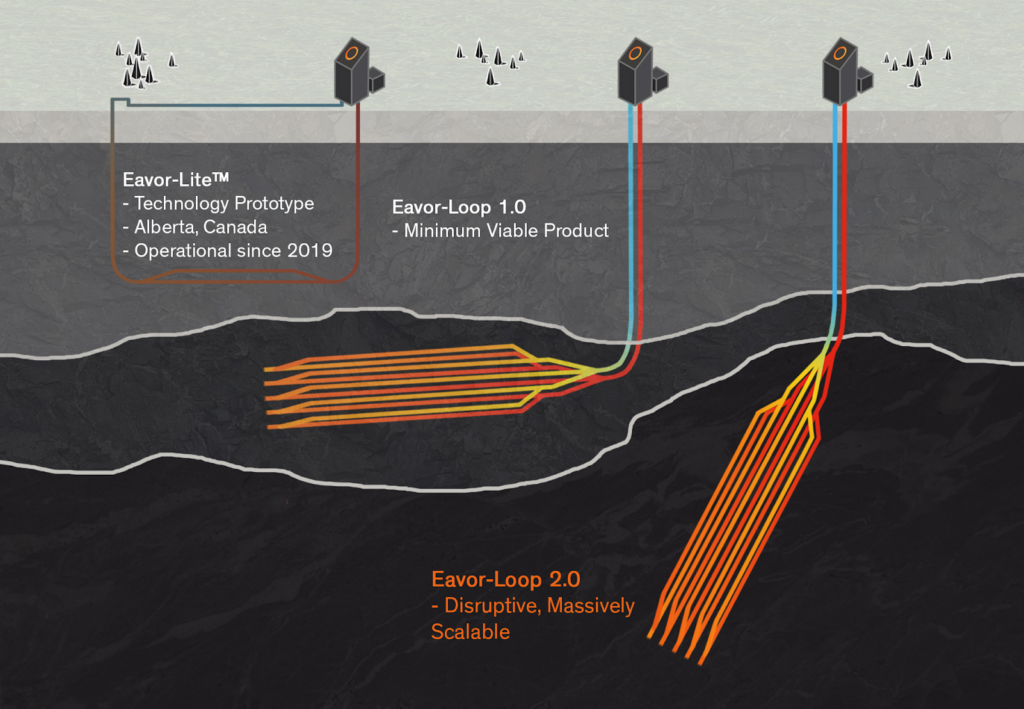
The main downside of closed-loop systems is that they cannot take advantage of the large surface area present in reservoirs. Rock does not conduct energy quickly, which is why the large amount of surface area in reservoirs is so important for conventional systems and EGS. There are hard constraints on the laws of conduction, limiting how much heat energy can be acquired by the system. But innovations may help. Eli Dourado wrote about a recently proposed design: “a solution to this is to use not just one horizontal segment, but many, like… radiator-style designs.” But these are expensive to drill and will require considerable iteration to solve.
Another concept for closed-loop systems is called a “heat roots” system:
“The idea is to drill a vertical well, and then form a hydraulic fracture[s] from that well that propagates downward into hotter rock. Then, a downhole heat exchanger is installed within the well. Free convection circulates fluid in the hydraulic fracture[s], bringing energy to the production well from the underlying formation.”
By using convection outside the system to bring heat to the closed loop, a heat roots system avoids the limitations of relying solely on conduction. However, heat root systems come with their own set of technical challenges:
“The flow conductivity of a single fracture will be insufficient to sustain the high flow rates needed for economic viability, nor will it access sufficient surface area to achieve long-term sustainability. [An economically viable project would require] 100s or 1000s of fractures, [and firms are] are experimenting with proppant to improve the flow rate achievable through those fractures…”
“… As hot water rises from the underlying part of the fracture (or as cool water sinks downward from the heat exchanger), it will equilibrate in temperature with the surrounding rock. This will prevent the high temperatures at the bottom of the fracture from reaching the top of the fracture, and vice-versa.”
The success of closed-loop systems relies on the industry’s ability to develop materials and designs with higher drilling efficiency and lower costs. Closed-loop has to contend with the physical limitation of low conductivity between rock and the system. Making the systems more efficient will require drilling more and deeper. The technological barrier for going deeper is drilling rigs that can perform consistently in granite, the hard basement rock that deep geothermal systems need to access. While this is a significant challenge, deep drilling has the potential for cost decreases on par with those the fracking industry created for sedimentary rock drilling.
Supercritical systems
The final frontier for next-gen geothermal is to develop super deep, supercritical technologies. Essentially, if you go deep enough, rocks get so hot that even water will go supercritical. This would have significant efficiency gains for electricity generation. The challenge is that getting to 500℃ rocks requires drilling to depths of around 20 km, much farther than current drilling technology allows (for reference, the Fervo well was drilled to a depth of around 2km).
One novel idea to drill deep is using millimeter-waves to vaporize hard basement granite. Qauise Energy is currently pioneering this technology. If successful, millimeter-wave drilling could start a new era of ultra-deep geothermal.
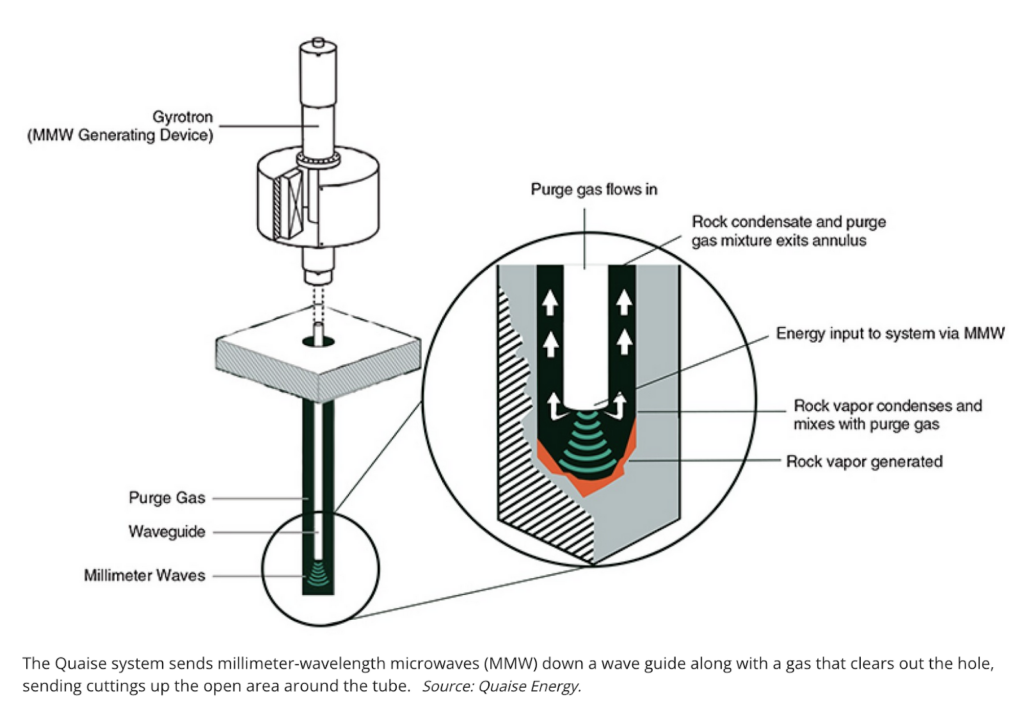
Super deep drilling is the most technologically uncertain, but offers significant upsides if developers can pull it off. Aside from the efficiency and production advantages, supercritical geothermal systems would be the ultimate geographic expansion; if you can drill deep enough, geothermal resources are available anywhere on the planet. Furthermore, the ability to access geothermal resources anywhere would allow geothermal companies to use existing or retired infrastructure, for example, by retrofitting retired coal plants. These sites are already connected to the grid, freeing geothermal companies from contending with transmission challenges.
Each approach to next-gen geothermal has its upsides and uncertainties. Rather than picking a favorite now, policy makers should embrace technological uncertainty and allow different companies to experiment.
Policy environment
To overcome technological challenges, geothermal companies need to “design, iterate, deploy and repeat.” Policymakers should be aiming to help companies shorten the innovation loop and iterate quicker. Companies need access to capital at early stages of technology development, and a regulatory environment that allows systems to be deployed and tried out in rapid succession. However, the current capital and regulatory environments pose significant barriers to rapid innovation.
Access to capital
The federal policies that supported the shale revolution essentially worked in three tranches. First, the federal government did its own research and development, and incentivized private R&D through cost-sharing and other demonstration projects. It also created market incentives, like demand supports and tax subsidies, that disproportionately benefited shale gas production over other production forms. And through the indirect mechanism of low interest rates, it increased access to affordable capital.
Today’s federal support for geothermal falls into three similar tranches. In the first tranche are a number of initiatives supporting geothermal projects throughout the country. The most targeted, the Geothermal Technologies Office (GTO) at DOE, supports the commercialization of EGS, advances geothermal exploration and drilling, promotes low-temperature geothermal systems, and researches barriers to exploration and development.
This year, GTO announced a $165 million grant for oil, gas, and geothermal professionals to leverage the skills of the oil and gas industry in geothermal deployment. While these kinds of investments drive innovation and efficiency for geothermal projects, next-gen geothermal will need to overcome scaling challenges to reach mass market adoption.
GTO has attempted to tackle those challenges through projects focused on scaling up EGS. Among these initiatives is the Frontier Observatory for Research in Geothermal Energy, or FORGE, a multi-million dollar field site dedicated to exploring new EGS technology and techniques. GTO is spearheading other initiatives like EGS Collab, focused on smaller-scale, experimental projects, and leading the department-wide Enhanced Geothermal Shot initiative, which aims to reduce the cost of EGS by 90% before 2035.
Another key player that has provided direct federal support for innovation in geothermal energy production is the Advanced Research Projects Agency-Energy (ARPA-E). ARPA-E has provided almost $40 million to 13 projects that advanced geothermal energy either directly or indirectly from their OPEN and Exploratory Topics programs. Recently ARPA-E awarded $8.2 million to develop next-gen geothermal technologies. ARPA-E has demonstrated success in funding geothermal technological advancements. In 2009, ARPA-E awarded Foro Energy $9.1 million to develop laser-mechanical drilling for geothermal energy. The award used a Technology Investment Agreement that required Foro to pay ARPA-E back in full with interest. Foro maintained ownership of its proprietary drilling technology, resulting in 45 patents. In addition, the company leveraged its relationship with ARPA-E to secure private financing and develop other DOE partnerships. ARPA-E’s experience crafting creative financing contracts for geothermal projects could be used to expand its investment in next-gen geothermal.
These are positive initiatives that could help advance next-generation geothermal development and deployment. Given that geothermal energy has the potential to meet more than 10% of the country’s electricity demand and reach 90 GW of generation capacity by 2050, it’s imperative that Congress continue advancing research and development initiatives at GTO and ARPA-E.
In the second tranche, incentives to accelerate the market for next-gen geothermal energy, the federal government has been less proactive. In the IRA, Congress expanded a handful of tax credits, traditionally reserved for other clean energy industries like wind and solar, to include geothermal. Specifically, Congress extended and modified the renewable electricity production tax credit (PTC) under Section 45 of the U.S. tax code and the investment tax credit (ITC) under Section 48 to include geothermal facilities, but the facilities must be constructed before 2025. The IRA created other manufacturing and production tax credits to be tech-neutral, and geothermal facilities could take advantage of these credits. These credits include the clean energy manufacturing credit (Section 48C), a clean energy production tax credit (Section 45Y) and an investment tax credit (Section 48E) for any facility that produces clean electricity. Through the production tax credit, geothermal power plants could receive $25 per megawatt-hour for the first 10 years of their operation.
While this may seem like a worthwhile incentive, the current cost of geothermal energy is $181 to $450 per megawatt-hour. Until the overall cost of production is reduced, a $25 incentive will not encourage new geothermal power plants to come online. The bulk of IRA tax credits that target geothermal projects expire within two years, and the new tax credits, while advantageous to geothermal production, do not target geothermal-specific projects. Without a policy that can make it relatively economical to produce energy, next-gen geothermal is unlikely to reach commercialization.
The third tranche, access to affordable debt, will be difficult to deliver today. While the shale revolution occurred during a zero-interest rate period, interest rates now are at their highest level in decades. However, subsidized credit is available from one federal office, the Loan Program Office (LPO) at DOE. The LPO holds a distinctive position in funding innovative clean energy projects that the private sector often considers too high-risk for investment, including next-gen geothermal. Under LPO’s Title 17 Innovative Energy Loan Guarantee Program (Section 1703), LPO has acknowledged that it can provide financing for next-gen geothermal energy projects, although the office has not yet supported any. Given LPO’s requirement for a reasonable prospect of repayment and the huge cost to produce next-gen geothermal at scale, meeting this requirement simply might not be possible in the current environment. Once next-gen geothermal energy can achieve cost reductions, or is more viable relative to other energy sources, LPO may be more inclined to make the investment.
To fully reach our climate goals and become a global leader on the path to decarbonization, public investment must be strategically centered on industries like next-gen geothermal. Federal research and development is critical, but success depends on broader financial and macroeconomic factors. Creative financing mechanisms and increased government incentives are needed to bridge the funding gap and encourage the development of groundbreaking next-gen geothermal energy solutions.
Regulatory environment
Geothermal companies need regulatory authorization that minimizes delays and increases learning by shortening the loop between research, development, and deployment. Unfortunately, the geothermal industry faces a particularly burdensome permitting process that limits the industry’s ability to learn and scale. The most significant geothermal resources are located in the American West, where most of the land is owned by the federal government.
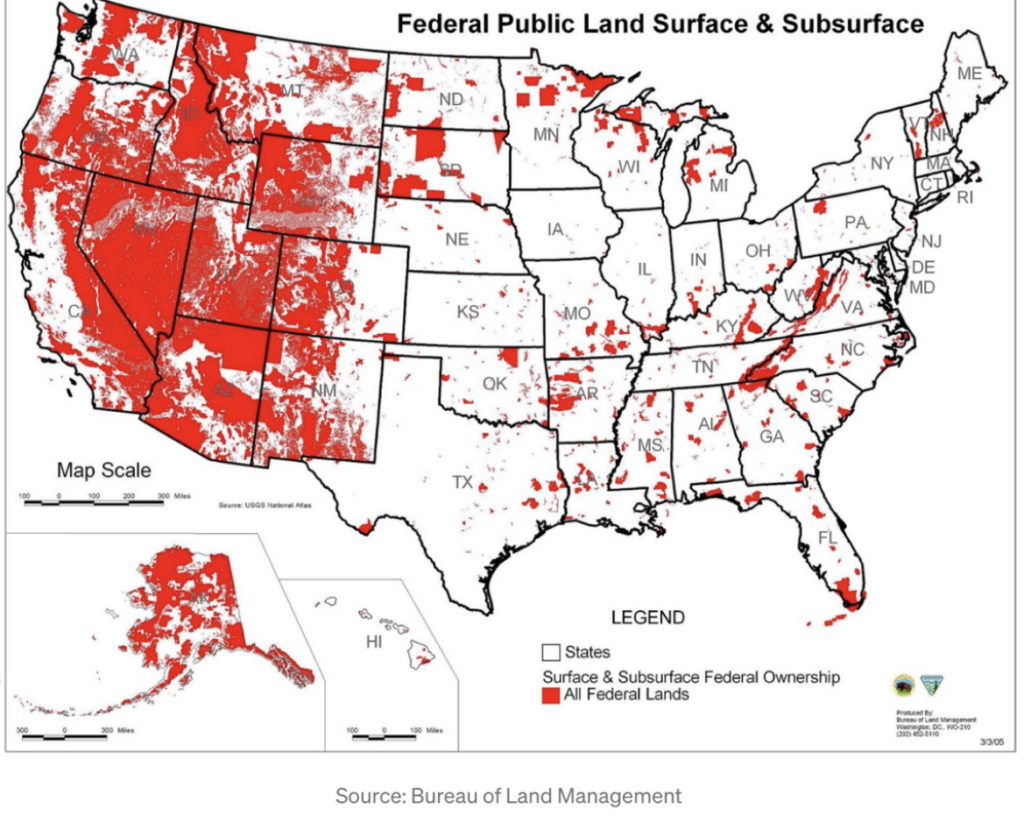
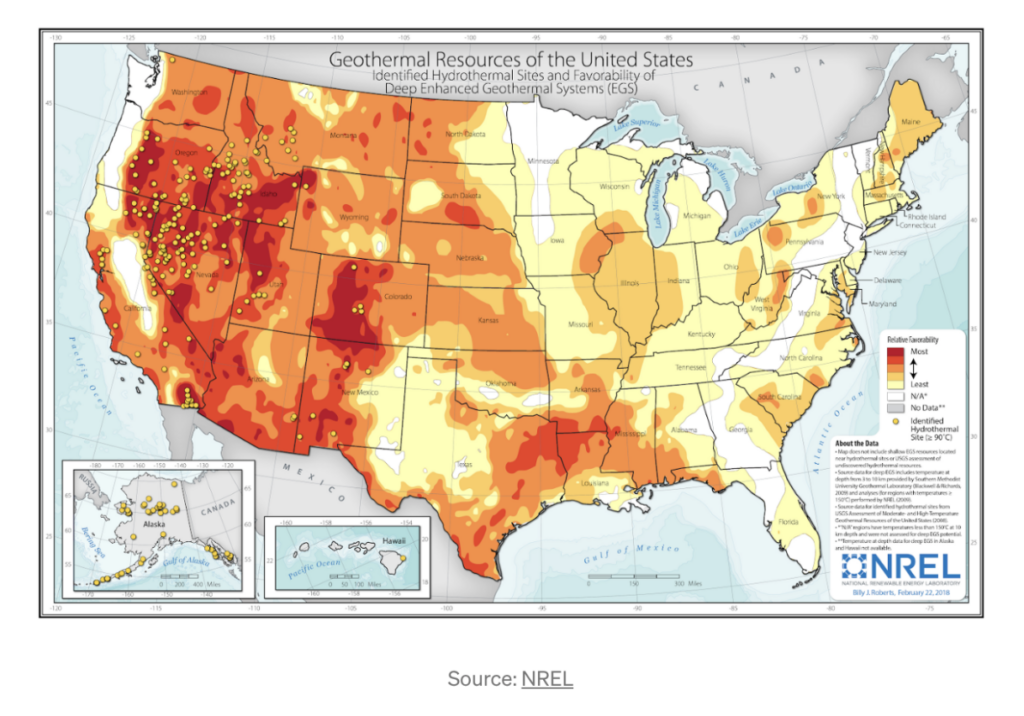
It could be effective for companies to iterate and experiment in the same region, much like the early days of shale development where the Eastern Gas Shales Project focused on the Appalachian Basin, or Mitchell’s focused on the Barnett Shale.
However, because geothermal resources are limited by geology and geography, developers are missing out on opportunities given that substantial geothermal resources are on federal land (which requires companies to run through the full gauntlet of federal leasing and permitting).
Geothermal is subjected to as many as six reviews under the National Environmental Policy Act:
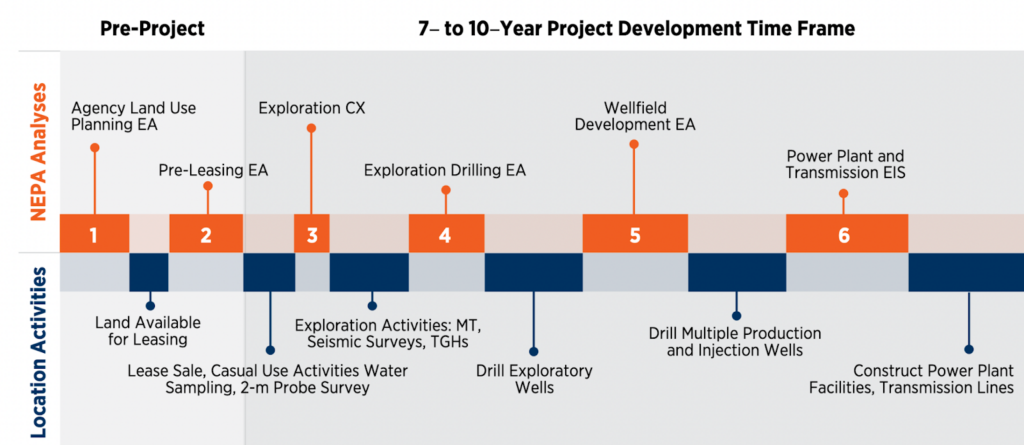
Geothermal developers are forced to complete a full environmental review before conducting exploration, and then another full review before final production development. This process is directly at odds with federal policy for oil and gas where new oil and gas wells are given a categorical exclusion for exploration.
Permitting adds a large uncertainty cost to geothermal development. Developers need to raise capital early in the planning process. But because of how long and uncertain the permitting process is, companies cannot provide investors assurance or even a final project plan until years into the project. Developers do not know when their project will come online, forcing them to factor in the potential loss of revenue and costs from permitting litigation. Permitting uncertainty gets stacked on top of the technological uncertainty that is inherent to companies pioneering next-gen technology. The result is that investors often shy away from making financial commitments, or significantly raise the price of capital.
Permitting is a clear drag on scaling geothermal. In 2022, multiple years after it began the permitting process, Ormat Technologies was forced to downsize its proposal to build a 60 MW conventional geothermal plant when litigious environmental groups got the Dixie Valley toad added to the endangered species list. Despite the significant downsizing, the project is still stuck in the permitting process.
Geothermal needs a policy update
Next-gen geothermal has enormous upside potential. The United States is home to some of the most accessible geothermal resources on the planet and has a world-leading tech innovation ecosystem. Next-gen geothermal could become an American-led industry that offers clean, firm, and practically unlimited energy.
However, the current capital and regulatory environments are headwinds, as cutting edge companies face high project uncertainty, expensive capital costs, and long permitting delays. In our final installment, we will look at the specific changes policymakers should make to accelerate the innovation loop and facilitate the growth of next-gen geothermal.